An evolution in the industry: Top trends for Space 2.0
StoryJune 22, 2022
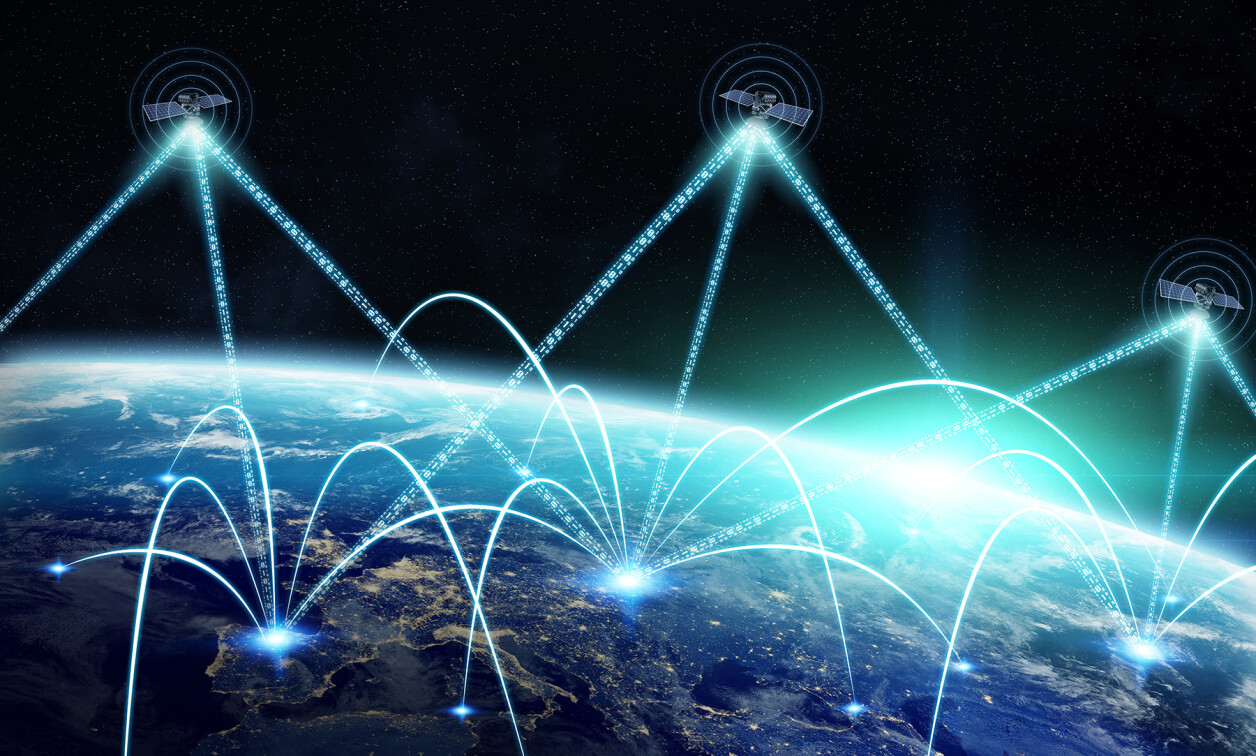
Space 2.0 represents a major shift in the development of defense and aerospace applications: With artificial intelligence (AI) applications moving aboard, systems must support higher processing and throughput capabilities. On-orbit processing requires an adaptive architecture so that systems can process, analyze, and reconfigure themselves to optimize performance and responsiveness. This, in turn, is driving innovation in organic packaging and reliability. Finally, to build these complex systems, engineers need greater design agility to accelerate development, maintain lower costs, and achieve faster time-to-launch.
There has never been a more exciting time to design for space. Developing and launching systems into space is no longer solely within the reach of governments. The innovation, agility, and vision of private enterprise are ushering in a whole new era: Space 2.0. The shape of space is expanding far beyond traditional defense and aerospace to an expansive range of practical – and profitable – applications.
Consider SpaceX’s low-Earth orbit constellation of satellites to provide broadband connectivity. Because these satellites require less fuel to get into orbit and are less expensive to launch, they can deliver value while having a shorter expected lifecycle of just four or five years. In this time, technology will have advanced and the next generation of satellites will be ready to replace them.
Emerging trends
The tremendous interest in low-Earth-orbit constellations goes well beyond simply connecting the world’s seven billion-plus people. There are countless applications possible with this technology. Using a traditional satellite can take up to a month to process an image. In contrast, a constellation of smaller craft can provide real-time imaging that can be used immediately to help firefighters on the ground, detect and track objects like planes using hyperspectral cameras and synthetic aperture radar, or transform how users navigate the planet, just to name a few examples.
Low-Earth-orbit satellites also can mean shorter missions, which reduces risk and costs. Using satellites in this way means a possible increase in the overall pace of innovation in space, moving to newer process nodes and packaging technologies much earlier. When the payload can be updated every five years instead of every 10 to 20 years, this enables mission specialists to do more with less each successive generation.
Among the major trends is the rise of on-orbit processing, which requires more compute and input/output (I/O) slots; this, in turn, is driving the move toward organic BGA [ball-grid array] packaging and away from legacy technologies like ceramic column-grid-attach solutions. Also seen: a sharp increase in development agility, resulting in faster evaluation, prototyping, and the launch of new technology. (Figure 1.)
[Figure 1 | On-orbit processing requires more compute and I/O, which means a shift in packaging requirements.]
Challenges of designing for space
Operating in space presents some of the most challenging barriers to design: First, the environment is extreme and unforgiving, and systems must be ruggedized and designed for no single point of failure. Downtime for maintenance is not an option in space. In addition, designers must deal with challenges such as:
- Limited downlink bandwidth: A satellite can capture a lot of data; however, the pipe to Earth isn’t wide enough to send it all back.
- Faster time to market: The window for launching new products is shrinking as development expands beyond traditional defense and aerospace applications.
- Designed for reuse: Space-based systems are no longer one-and-done; they must now be platforms whose IP can be reused across multiple missions.
- Low latency and high bandwidth: For broadband communications to be viable, the system must have minimal latency with seamless and reliable connectivity. (Figure 2.)
[Figure 2 | The challenges of designing for space are detailed.]
Machine learning in space
The foundation of addressing these design challenges is to offload processing from the ground station and bring it on-board. Rather than sending data and images to Earth for processing – and introducing all the latency associated with this – satellites will process data themselves and send information about what that data means instead. This requires satellites to support AI capabilities in orbit, including object detection and image classification, to start.
A key part of making on-orbit processing viable is understanding that AI is an ever-changing field of research and that machine learning (ML) models require continual optimization. First, ML models can adapt over time to become faster and more accurate. Second, the algorithms themselves change as new breakthroughs are made. Thus, space-based systems need a flexible and adaptive architecture that can change models and algorithms “on the fly.”
Because ML is involved, programmable software is not enough. ML is compute-intensive and requires hardware acceleration to provide real-time responsiveness. When the algorithms change, the hardware needed to accelerate the algorithms change as well. Thus, an adaptive platform requires a combination of configurable software and hardware that can update in concert with each other. In short, to support on-orbit processing, systems need to be able to process, analyze, and reconfigure from the architecture up through to the application code.
Moving toward organic packaging
Being able to deliver reliable system components that will operate during the long mission life needed and the extreme environments found in space require a completely different level of design, manufacturing, and testing. Quality control must work with design teams from the very start to achieve the levels of reliability required by the government.
For example, Six Sigma, an established and reliable leader in the defense and aerospace industry for over 30 years, is the sole supplier of solder column attachment to ceramic-grid-array packages, primarily used in space applications. While the government has actively sought out a second source, the processes and expertise required to provide the world-class reliability offered by Six Sigma are so rigorous that, to date, no other supplier has been able to achieve certification.
As the industry moves toward new process nodes like 7 nm technology, the dies are too large for legacy space-grade packaging and techniques like solder column attachment. Simply put, the processing requirements for on-orbit AI won’t fit anymore. There’s also the significant increase in I/O to consider.
As a result, the industry is beginning to move away from legacy packaging and to organic packing and flip-chip packaging for space-grade products. In addition to being able to support the larger die size and I/O needed, organic packaging reliability has been proven in the commercial market and has a much wider ecosystem of support.
Of course, there are still challenges to overcome: Space development will not shift overnight. It takes years to qualify space-grade products, and the many legacy ecosystems in place will continue to need support. However, the defense sector is interested in having access to the latest technology, and the players understand that innovation means change.
Continued innovation in space-based design and systems
The defense and aerospace industries – as well as any company considering space-based applications – need technology that can provide the necessary performance, adaptability, and reliability for Space 2.0 applications. New technology alone is not enough, however. As systems become more complex, the difficulty in integrating components becomes more challenging. Even evaluating a simple ML platform can take weeks when developers must integrate components from multiple vendors themselves.
It’s critical to understand the demanding requirements developers face while building reliable systems for space.
True innovation and on-orbit reconfigurability will be possible with:
- 2.5/3D die integration and packaging technology
- Chiplet and chip-to-chip (C2C) interconnect technology
- AI engines and domain-specific architectures
- Next-generation routing to eliminate congestion
- ASIC-like clocking with flexible clock placement and skew balancing
- Intelligent 3D analytical placing tools to optimize timing, congestion, and wire length
- Soft-core processors supported by tools for ML-centric applications
Space 2.0 promises an exciting future. The ability of the private sector to launch its own systems brings new vision to the industry. On-orbit processing will extend the capabilities of space-based systems into viable commercial applications that improve quality of life around the world. True unlimited on-orbit reconfigurability provides the software and hardware flexibility these systems need to implement and accelerate real-time AI capabilities. The move to organic packaging will enable the industry to onboard the processing and I/O required for next-generation systems. OEMs will enjoy the many benefits of design agility as it becomes easier to evaluate, design, adapt, and reuse space-based IP.
Inderjit Singh is the Senior Director of Assembly & Packaging Engineering Group at AMD. He has been in this role for the last 11 years as part of the Adaptive and Embedded Computing Group (formerly Xilinx). He has more than 31 years of assembly, manufacturing, package development, design, reliability, and chip-to-package interaction experiences. He holds a bachelor of applied science degree, majoring in applied physics, from University Science Malaysia.
Minal Sawant is the Director for Aerospace & Defense Products at AMD. As part of the Adaptive and Embedded Computing Group (formerly Xilinx), she is responsible for driving the business strategy for AMD A&D solutions and drives enablement of new-generation platforms and architectures. Minal has supported defense, aerospace, and high-reliability markets for over 20 years. Minal holds a master’s degree in electrical engineering from University of Oklahoma.
AMD https://www.amd.com/en