Riding the waves: How RF tech is supercharging military 5G
StoryOctober 08, 2024
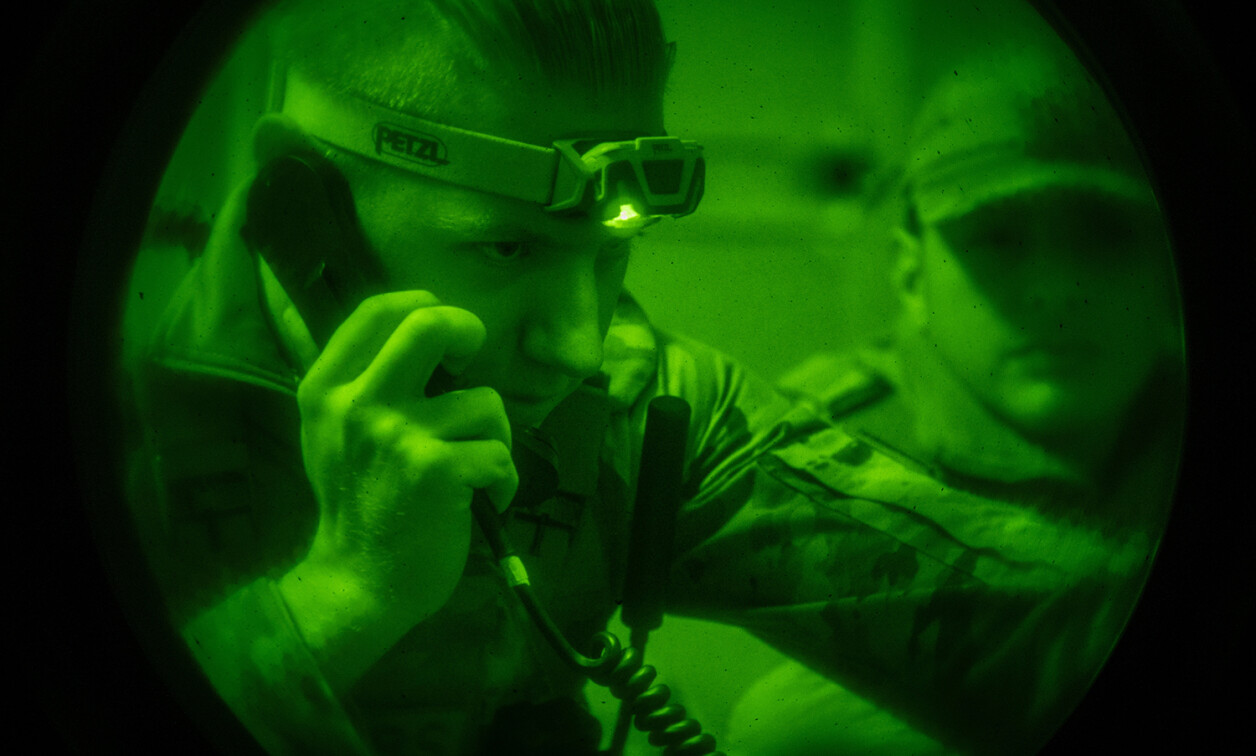
In the near future, soldiers tap smartwatches to summon 3D maps, drone swarms execute perfect reconnaissance missions, and autonomous vehicle fleets navigate treacherous terrain in perfect sync. This is the promise of 5G technology: boosting military communications with unprecedented speed, capacity, and reliability.
In order for the battlefield 5G revolution to happen, it needs to realize rapid advancement of radio frequency (RF) and microwave technologies. From miniature antennas that bend signals like light to power amplifiers that pack a lot of equipment into a featherweight package, these RF innovations are the unsung heroes of the 5G era.
Yet questions abound: How can engineers fit more power into increasingly smaller RF components? How do they ensure these tightly packed components work well together under tough conditions? And how can the industry push the boundaries of power amplifier technology to meet the ever-growing demands of the military?
RF and microwave: The invisible force behind 5G
RF and microwave technologies make it possible to send messages across the globe on a 5G network. These technologies act as the invisible highways that carry vast amounts of data at unprecedented speeds and with minimal delay, enabling everything from real-time video streaming to remote robotic movements.
5G networks rely on electromagnetic (EM) signals from about 450 MHz to higher than 50 GHz in order to communicate voice, data, images, and videos across significant distances without wires, says Art Aguayo, technical business development executive at Benchmark (Tempe, Arizona).
“Since 5G networks employ wider instantaneous bandwidths compared to previous wireless cellular network generations, they can send and receive more information securely and simultaneously, at higher data rates and lower latency than earlier cellular generations,” he continues. “The technology is well-suited for critical communications for armed forces in areas with access to 5G infrastructure or cells.” (Figure 1.)
[Figure 1 | Benchmark Lark Technology mmW-STL bandpass filters can be customized for frequency bands as high as 40 GHz in a SWaP-optimized package.]
5G networks can send and receive much more information at once, and do it faster and more securely – like upgrading from a country road to a superhighway with express lanes. This supercharged network isn’t just one big antenna, however; it’s a complex web of different-sized cells working together.
“Macro base stations can talk directly to the user equipment cell phones or can talk to the small cells, which talk to the user equipment mobile device providing the last-mile connectivity,” says Baljit Chandhoke, product manager of RF products at Microchip Technology (Chandler, Arizona).
In some ways, it’s like a relay race: The macro base station hands off the data to smaller cells that carry it the final stretch to a device. These smaller cells come in different types: Femtocells are like personal mini-towers for an individual location, while picocells cover up to 300 meters and can support 100 users, Chandhoke notes.
This technology isn’t just theoretical – it’s being used today, says Kerem Ok, product line director at Analog Devices (Wilmington, Massachusetts).
Operators are “taking advantage of improvements in RF linearity and power efficiency to increase the range and throughput of their radios while reducing the cost of installation, maintenance, and running the network,” he says.
The result is a flexible, powerful network that can provide lightning-fast communication whether you’re in the heart of a city or the middle of nowhere. For the military, this means unprecedented connectivity and data-sharing capabilities, potentially changing everything from logistics to battlefield tactics.
Advancements in RF and microwave tech
Imagine shrinking a bulky radio into something that fits in your pocket, but works even better. That’s what industry is doing right now, Aguayo says. “Military electronic applications have stressed the need for solutions with smaller size, weight, and power (SWaP) for enhanced mobility and portability,” he notes.
Scientists are also tapping into higher frequency bands, which is like opening up a new lane on a crowded highway.
“Development of affordable millimeter-wave components is simplifying extension of 5G networks into the millimeter-wave frequency spectrum with its wide available bandwidths,” Aguayo says.
These new millimeter-wave 5G communications solutions are “substantially increasing how much information can be shared in support of real-time decision-making,” Chandhoke says.
There are many applications for these solutions as well. “5G enables a variety of smart-warehouse, telemedicine, command-and-control data gathering, augmented-reality displays, virtual-reality solutions for remote vehicle operation in air, land, and sea missions,” he says.
Engineers are not just improving existing tech – they’re completely rethinking how it’s built, Ok says. He predicts big changes in how these systems are designed over the next few years. (Figure 2.)
[Figure 2 | Analog Devices’ ADAR4000 2 to 18 GHz transmit unit with true time delay and digital step attenuator, offering 31.8 dB gain adjustment and 508 ps time delay for precise beamforming in phased-array systems.]
Even large defense contractors that do not directly make 5G parts are getting in on this technology. Raytheon, for example, is working on related technologies like advanced antennas and power amplifiers.
“There are some commonalities between RF components that are used in commercial deployments and defense systems that Raytheon manufactures,” the company said in a statement. “In general, these include phased-array systems that are enabled by silicon beamformer technology, high-power and high-efficiency GaN [gallium nitride] power amplifiers, advanced packaging, and thermal management.”
Designing 5G RF components
When designing RF components for 5G systems, engineers are essentially trying to build a microscopic city with perfect traffic flow in the middle of an earthquake. As the industry pushes into higher and higher frequency ranges, a whole new set of issues can arise. In particular, the military wants industry to find ways to cram more power into tinier spaces, Aguayo says.
“As 5G expands its use of frequency spectrum into the millimeter-wave range, some of the main challenges include providing increased functionality and performance in smaller packages, such as integrated circuits (ICs) and densely packed printed circuit boards (PCBs),” he adds.
But it’s not just about making things smaller. These components also need to work with each other in tough conditions, Aguayo continues. “Because of such close placement, components and devices must be carefully specified for electrical, mechanical, and thermal compatibility over extended operating periods and under hostile environmental conditions.”
Another problem is that higher-frequency signals don’t travel far and struggle to get through walls, Aguayo notes. This means 5G networks need more base stations, especially in built-up areas.
Also when designing phased arrays, a key part of 5G systems, it is difficult to get them to work in sync, Ok says. “Phased arrays are notoriously difficult to get right, especially for larger array sizes that need to transmit complex waveforms like 64/256QAM,” he says.
"A multivendor approach exposes engineers to additional testing and qualification demands as key pieces of IP need to work in harmony to get the best performance possible in these complex systems," Ok says.
Power amplifiers: boosting 5G performance
Improving 5G performance means more power, accomplishing that without bulky power equipment is difficult. That’s the challenge facing engineers working on power amplifiers (PAs) for 5G systems. These tiny powerhouses are the muscle that makes 5G signals strong enough to move across vast distances, and they’re a major focus for engineers.
“Because military users seek reduced SWaP, power amplifiers for 5G networks must be designed with increased power density – providing increased gain and output power from smaller packages,” Aguayo says.
But it’s not just about raw power; amplifiers need to be smart, too.
“Higher efficiency is also a key requirement since 5G PAs are needed to boost often low-level signals, such as from a phased-array antenna, under what may be hostile or degraded operating conditions,” he says.
The secret is gallium nitride on silicon carbide (GaN on SiC). This super-material is how these amplifiers achieve much more power than their small size suggests, Chandhoke says. (Figure 3.)
GaN on SiC power amplifiers can operate at high frequencies in the Ka-, Ku-band from 12 GHz to 40 GHz and have broad bandwidths, he explains. This setup results in “high gain with better thermal properties meeting the requirements of 5G applications,” Chandhoke adds.
[Figure 3 | Microchip’s ICP2840 GaN on SiC power amplifier operating in the Ka-band used in satellite communications and 5G mmWave.]
As with any cutting-edge tech, there are hurdles. Ok points out that as chips shrink to pack in more features, it gets harder to maintain performance – like trying to fit a V-8 engine into a go-kart.
To cope with the smaller package sizes, researchers are coming up with new designs to squeeze every ounce of efficiency out of these amplifiers. Ok says he believes these innovations are key to building 5G systems that can compete on the global stage.