Next generation of electronic warfare systems to feature enhanced RF and microwave hardware
StoryFebruary 15, 2023
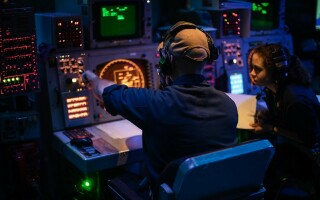
The proliferation of new, more lethal threats and the need for improved situational awareness in the electromagnetic spectrum fuel more sophisticated radio frequency (RF) and microwave designs. Meanwhile, open architecture efforts are enabling faster adoption of techbnlogy and lowering long-term costs.
As radar and electronic warfare (EW) system providers continue to reduce size, weight, and power (SWaP) while increasing performance demands, this move puts more and more pressure on RF and microwave component designers to pack more capability in ever-shrinking footprints.
Supporting a sensor chain that stretches across multiple nodes on a battlefield – from manpack radios, to uncrewed ground platforms, to phased array radars – requires high-speed and low-latency EW systems and innovative solutions from industry.
“We’re not only seeing tremendous advocacy and strong defense budgets but also cutting-edge technology we’ve had for decades being taken out of the lab and put into operation,” says Erin Kocourek, vice president of advanced technology and growth at CAES (Arlington, Virginia).
This direction is leading to higher speeds, greater maneuverability, increased range, and the use of more exotic materials for better thermal management, she adds.
Demand for multifunction capabilities is also increasing as warfighters face more complex threats, says John Cowles, senior director of technology for aerospace and defense at Analog Devices (Wilmington, Massachusetts). “What military customers are looking for is this idea of a receiver and transmitter that can do anything, a multifunction platform that can serve EW, radar, and telecom at the same time.”
Reduced size, increased performance
Requirements for both EW and radar systems include increased performance systems in smaller form factors.
“There is always pressure to do more in a slot,” says Mark Littlefield, senior manager of embedded computing products for Elma Electronic (Fremont, California) “The latest VITA 65.1 revision (2021) has aperture fill blocks that have 10, 14, 20, and even 31 RF contacts. Clearly, folks are cramming more and more functionality into each VPX slot.” (Figure 1.)
[Figure 1 | Elma’s 3U 12-slot backplane that aligns with The Open Group Sensor Open Systems Architecture (SOSA) Technical Standard 1.0 is an update to Elma’s original CMOSS [C5ISR/Electronic Warfare Modular Open Suite of Standards] reference backplane supporting the U.S. Army’s latest open-standards requirements. The new 3U OpenVPX backplane offers a mix of plug-in card (PIC) slots that enable complex, high-speed signal processing and system development, supporting up to 100 Gbit Ethernet.]
There’s no single solution to optimizing for SWaP, notes Tony Capello, chief technologist at Mercury Systems (Andover, Massachusetts). “Instead, we consider design trade-offs throughout the development process. We carefully select the architecture to meet performance in the smallest size possible. Additionally, we work at chip scale to combine devices in much smaller space,” Capello says.
Even with these tradeoffs, design challenges persist: cooling the electronics, maintaining signal integrity, and avoiding unwanted coupling while squeezing more components into smaller spaces.
According to Cowles, apart from SWaP requirements, the “big thing is cooling, power, and dissipation. Even if, by contacting things, you maintain the efficiency, you still have to get the power and you still have to get that heat out.” Analog Devices supplies the ADRV9002, which is a high-performance, linear, dynamic range transceiver designed for providing performance and power consumption system optimization. (Figure 2.)
[Figure 2 | The Analog Devices ADRV9002 is a high-performance linear dynamic range transceiver designed to enable optimization of performance and power consumption.]
Kocourek points out that wide-bandwidth low-noise amplifiers (LNAs) and mixers can offer increased bandwidths and smaller size, weight, power, and cost (SWaP-C), enabling more users to implement EW receivers to handle electronic-support (ES), electronic-intelligence (ELINT), and signals-intelligence (SIGINT) applications.
Digitizing the signal earlier on in the sensor chain via advancements in semiconductor design also enables reduction in system size and increased product flexibility.
Capello says that Mercury is working with semiconductor companies to integrate their die (for example, data converters or FPGAs [field-programmable gate arrays]) at chipscale in extremely compact footprints. “However, integrating these devices on open architecture boards still requires compact and high-performance microwave components,” he adds.
Distributed, networked solutions across multiple military platforms can help solve SWaP challenges. Direct-to-digital implementations will also show promise in the next few years, says Dr. Larry Scally, vice president of CAES Missiles AT&E (Colorado Springs, Colorado). “Direct RF sampling adds a lot of capabilities to EW, but also adds a lot of power. For example, digital signal processing with ADCs and DACs [analog-to-digital converters and digital-to-analog converters] running at higher speeds requires much more power, size, and weight than RF components. Like everything in engineering, there is no free lunch – it is a trade-off for SWaP-C, programmability, and performance,” he explains.
In terms of reduced-form-factor solutions, CAES offers a new 0.5 to 18 GHz SOSA [Sensor Open Systems Architecture] aligned 3U ultrawide-bandwidth RF converter which provides two channels of high-speed tuning in a single slot with the local oscillator and preselection for EW, ELINT, and electronic support, surveillance, and attack systems.
Modifying architectures
The design and architecture for RF and microwave hardware has been modified over the last years to improve such systems.
Sensor architecture implementation is changing in order to simplify the RF front end, says Ben Annino, systems and applications director for aerospace and defense at Analog Devices. “Data converters can now directly sample much wider swaths of bandwidth. Years ago, you could do tens of MHz, it moved up to hundreds of MHz, and now you are able to do GHz, multiple GHz, instantaneous bandwidth all at once. This is because data converters have moved into finer silicon.”
The digitization process is also changing the architecture and “the combination of noise, distortion gain, and power dissipation evolved to be different from what they used to be,” Cowles says
Efficiency challenges
Improving the efficiencies of components through the use of different materials and processes can also help reduce SWaP. Raytheon Missiles & Defense’s gallium nitride (GaN) program focuses on advancing the efficiency of the company’s GaN manufacturing process to improve yield and reduce cost, while generating more RF power.
Raytheon engineers have been working on new materials that offer higher current and voltage transistors, which could result in more efficient devices and offer new trade spaces to system designers for power per element vs. aperture size.
“At the subsystem level of an EW or radar system, RF microelectronics like GaN typically dominate overall power consumption and cost. At the system level, a GaN device with higher efficiency results in extended range, enhanced sensitivity, and/or reduced power consumption,” says Matt Tyhach, director of next generation sensors and microelectronics/advanced technology at Raytheon Missiles & Defense (Tucson, Arizona). (Figure 3.)
[Figure 3 | Raytheon’s gallium nitride (GaN) offerings can enable extended range, enhanced sensitivity, and reduced power consumption.]
GaN is part of several U.S. Department of Defense (DoD) weapons programs, including the Enterprise Air Surveillance Radar (EASR); the Guidance Enhanced Missile Tactical Ballistic Missile (GEM-T), one of the Patriot missile variants; and the Lower Tier Air and Missile Defense Sensor (LTAMDS).
MOSA strategies
Even with those advancements, EW system designers continue to seek additional improvements that affordably enable future upgrades without significant impacts to the rest of the system. Such interoperability efforts fall under the DoD’s modular open systems approach (MOSA) mandate which calls for implementing MOSA strategies like SOSA and the OpenVPX standard. These MOSA examples and others enable the use of nonproprietary interfaces in designs.
“The trend towards open system architectures is only increasing. From the end-user perspective, they need to deploy new technology as soon as it’s available,” Mercury’s Capello points out.
Mercury offers multiple OpenVPX and SOSA aligned solutions, including the DRF3182 3U OpenVPX board. Designed for high-performance applications with SWaP constraints, it is a 4Rx/4Tx, ultra-high-speed data converter ideal for directly digitizing HF signals in radar, communications, and EW systems. (Figure 4.)
[Figure 4 | The Mercury DRF3182 3U OpenVPX board is a 4Rx/4Tx, ultra-high-speed data converter ideal for directly digitizing HF signals in radar, communications, and EW systems.]
From Kocourek’s perspective, the requirements for open system standards are also helping to bring adaptability: “More EW systems are adding requirements for standardization with a clear definition of RF module and sensor system form factors, pinouts, I/O formats, and power requirements.”
Other open architecture benefits include shortening development cycles and reducing long-term life cycle costs. Moreover, enabling the operation of sensors and technologies from different suppliers would also allow expanding the vendor base. “Enabling multiple sources for the same subsystem or components would make the system less impacted by potential supply-chain issues,” Tyhach adds.
SIDEBAR
Radar and EW: What’s similar/what’s different
Electronic warfare (EW) and radar differ slightly in the RF waveforms used and how they interact with the rest of the system. Electronic warfare typically operates via continuous wave (CW), which is on all the time. Radar waveforms, in contrast, are pulsed, which enables amplifiers to cool down between pulses.
Radar bandwidths are also generally smaller – even though there is a trend to move wider – and require higher peak powers. In the case of EW, it uses a much wider band, with its electronics needing to transmit and receive power over wider frequency ranges.
“These differences can prove challenging, particularly in EW thermal hardware design. Radar requires higher peak powers where RF peak voltages, including power storage, need to be properly accounted for to generate the pulses,” says Matt Tyhach, director of next-generation sensors and microelectronics/advanced technology at Raytheon Missiles & Defense (Tucson, Arizona).
There is “a big difference” between those types of systems because for radars, “you are transmitting a known waveform and then that waveform is coming back,” and the operator has a prior knowledge of what it is,” says Ben Annino, systems and applications director for aerospace and defense at Analog Devices (Wilmington, Massachusetts). “For EW, you don't know what you're trying to detect, and what you're trying to detect can also be trying to kill you. So, you need to have basically continuous monitoring [of a] higher RF spectrum.”
EW systems “have to react very, very quickly to what's coming in and decide what it is, and say what to do about it,” says John Cowles, senior director of technology for aerospace and defense at Analog Devices.
Although radars and EW solutions perform different missions, they have common needs. Both require high-frequency operation in the GHz realm, defense-grade reliability, and novel heat-rejection approaches. Lower latency, higher speed, and reduced form factor are also necessary for each.
David Caserza, manager of embedded computing architects at Elma Electronic (Fremont, California), says that another similarity is the fact that it is necessary for both radar and EW to produce backplanes and chassis that can accommodate the RF signals/connectors/cabling.
“For the fighter, both EW and radar need high-end processing back ends to deal with the additional number of signals and threats,” says Erin Kocourek, vice president of advanced technology and growth at CAES (Arlington, Virginia). In terms of solutions, CAES offers the USTAR C-Band radar that provides autonomous collision avoidance for both manned and unmanned airborne platforms. (Sidebar Figure 1.)
[Sidebar Figure 1 | The CAES USTAR C-band radar promises autonomous collision avoidance for both manned and unmanned airborne platforms.]
Going forward
There is increased demand for next-generation active electronically scanned array (AESA) systems, which require “extremely compact RF/digital hardware at each antenna element as well as high-performance microwave subassemblies throughout,” says Tony Capello, chief technologist at Mercury Systems (Andover, Massachusetts).
The push toward operating on even broader bands and moving to higher frequencies is also putting “a lot of pressure on maintaining efficiency and [controlling] cost,” Cowles adds.