Lower-SWaP LOS radios help secure battlefields
StoryMarch 21, 2023
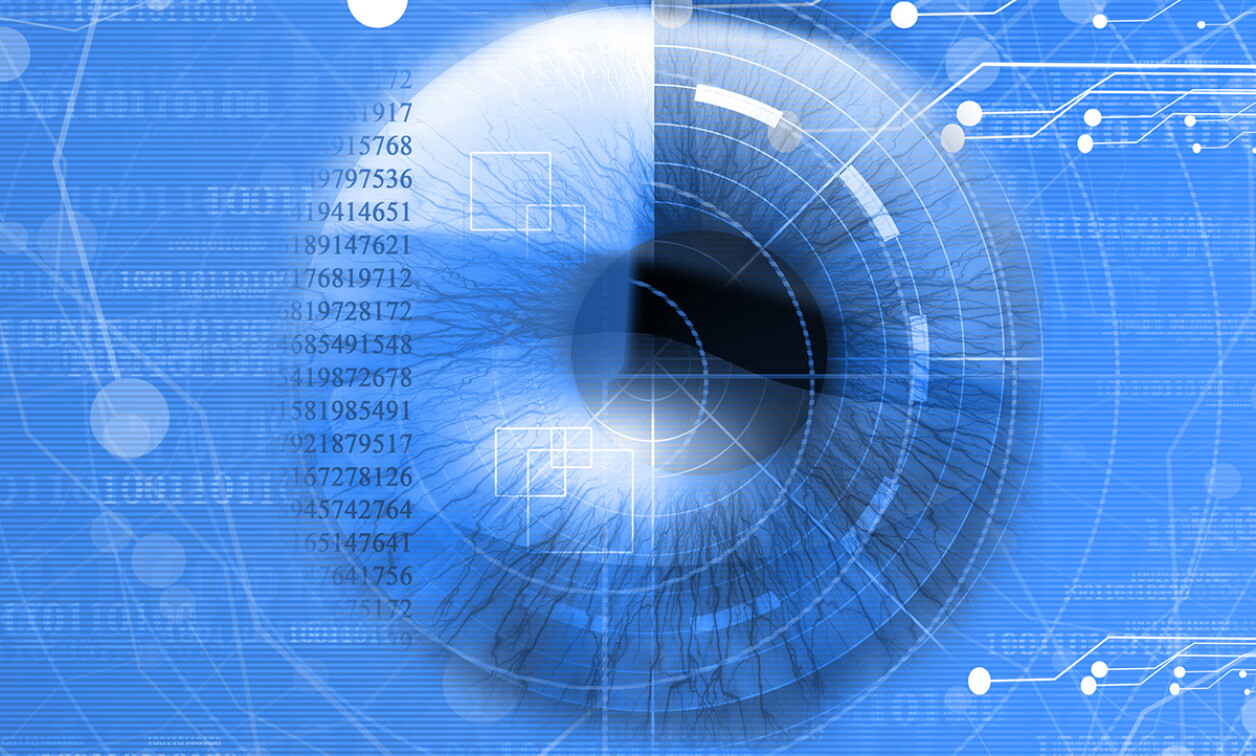
The ongoing need for reduced size, weight, and power (SWaP) is driving the development of secure specialized line-of-sight (LOS) radios used on the battlefield. These radios demand increased functionality in smaller packages, even in single-board solutions, used in body-worn equipment, on-field IoT sensors, or wireless sensors on unmanned aerial or ground vehicles.
Communications equipment has evolved as much as any systems designed for the modern electronic battlefield, with the reliability and security of radio links often significantly impacting the success – or failure – of a tactical mission. Communications signals are transmitted and received by systems at sea, aboard aircraft, and even via satellite communications (satcom) links formed with orbiting satellites.
Often, however, the most critical commands or the sharing of intelligence comes by way of short-range terrestrial, line-of-sight (LOS) radio links that must send and receive signals with the confidence that an adversary is not intercepting or jamming those signals. Known as “low probability of interference, low probability of detection” (LPI/LPD) and antijam radios, they are essential in establishing the covert, reliable communications needed to exchange voice, data, and video communications among troops in the field without alerting an adversary to the warfighters’ presence.
Tactical radio equipment operates within crowded spectrum environments where the electromagnetic (EM) signature is closely mapped and monitored by wideband receivers such as those found in electronic warfare (EW) and electronic intelligence (ELINT) systems. Omnidirectional (or nondirectional) radio signals are prime candidates for detection since their beams cover wide areas. The U.S. Department of Defense (DoD) has confirmed plans to employ the increasingly extensive capabilities of 5G cellular wireless communications networks on the battlefield. This as-yet-to-be-erected millimeter-wave (mmWave) frequency portion of its infrastructure will provide the generous amounts of spectrum needed for high-speed-data transfers and real-time in-field video streaming (albeit at shorter propagation distances than the longer wavelengths of 5G’s lower frequencies).
Once necessary infrastructure is erected (Fig. 1), tactical use of 5G wireless cellular networks has the many benefits of a full-fledged, multi-function communications network, supporting manned and unmanned interoperations and low-latency communications for mission-critical applications. But 5G communications networks are not secure and can be disrupted by EW and cyberattacks. In addition, for tactical use, 5G networks must be adapted to existing battlefield communications formats, including point-to-point LOS radios and satellite-based communications systems. (Figure 1.)
[Figure 1 | U.S. Air Force personnel place protection around the inner ducts for the fiber-optic cables connected to a 5G cell tower at Ramstein Air Base in Germany during a 2021 operation. U.S. Air Force photo by Airman Jared Lovett.]
While its use of mmWave spectrum may be unique on the battlefield, an EW receiver – or even a spectrum analyzer with directional antenna in the signal path – can detect and intercept/record the signals for analysis; in other words, to apply machine learning [ML] algorithms to the development of jamming signals. Jamming signals at mmWave frequencies can be cost-effectively produced through the multiplication of lower-frequency signals, like those from an RF/microwave signal or function generator).
Pursuing LPI/LPD operation
Secure battlefield communications have long been accomplished by two-way LOS radios. They lack the capacity for almost unlimited number of users of a network like 5G but do not require the large infrastructure of a wireless network. Communications of voice, data, text, or video are accomplished between two points on the battlefield with mobile equipment. Modern LOS radio equipment is compact and efficient enough for a wide range of embedded applications, including in personnel-worn radios, links with in-field Internet of Things (IoT) sensors, and wireless sensors on unmanned aerial systems (UASs) or unmanned ground vehicles (UGVs).
Because two-way LOS radios are fully mobile, the signal path can change at any time, decreasing the probability of detection and interception. LOS radios designed to meet mobile ad hoc networking (MANET) requirements also provide networking capability among multiple LOS radios when needed, although MANET interoperability must be fail-safe to support reliable battlefield-networking communications. In addition, practical tactical radio solutions should be backwards-compatible to enable interoperability with radio equipment in the field.
The transmit-power levels of portable LOS tactical radios are well-controlled to minimize EM levels that might be detected by an adversary. Of course, limiting transmit power leads to a trade-off in performance: Higher power levels help overcome propagation attenuation in the signal path from such factors as foliage, high humidity, or reflections from buildings in populated areas. Higher power levels support the high-throughput performance needed for clear voice communications and fast data-transfer rates. But higher power levels are also easier for enemy receivers to detect and track, even if transmit signals result in reflected beams that can be detected. Analysis of the variations in the angles of reflection can lead to identifying the main signal path. At lower transmit-power levels, those reflections may be too low in level to be detected.
The usable communications range of any LOS radio can be determined by its transmit power and its transmit power density which describes how the transmit power is distributed across the channel bandwidth. Radio transmitters with low transmit-power density are ideal since they are difficult to detect, especially in crowded spectrum. Provided the trans-mit power is sufficient to overcome signal-path losses over a required operating distance to the receiver, low transmit-power density is a desirable characteristic for radios designed for LPI/LPD functionality.
To increase effectiveness in extremely hostile conditions, LPI/LPD radios are designed with high resistance to jamming signals. Often this is achieved through secondary receiver circuitry capable of providing details about the jamming signals for instantaneous development of an antijam algorithm. An antijam algorithm can model a form of inverse LPI/LPD radio waveform for cancellation of any jamming signal components received with the desired carrier signals in the LPI/LPD’s primary receiver stage. Additional antijam techniques include the use of software-defined radio (SDR) architectures to signal the radios in a link to switch to a channel that is not occupied by jamming signals or to employ a frequency-hopping algorithm to move the carrier signal within a total bandwidth that exceeds the limits of the jamming signals.
In a crowded EM spectrum with multiple signals occupying wide and narrow bandwidths, distinctive waveform characteristics can make it easier to identify a radio that has been recently added to the operating environment. For this reason, unit-to-unit consistency of performance is critical for successful LPI/LPD radio operation. Lack of uniformity enables adversaries to focus on a single radio and use its signals for geolocation of a warfighter using feature detectors, for example a signal analyzer or oscilloscope programmed to identify waveforms that fall outside a given feature limit such as larger-than-average amplitude variations.
For extended-range, multiple-user operation, LOS radios require some infrastructure like antennas and towers capable of relaying in-field signals to a base station or control center, but this auxiliary equipment is not on the scale of the large towers and repeaters that comprise wireless cellular communications networks such as 5G systems. In addition, when fully functional, 5G will operate within two portions of frequency spectrum, commonly referred to as the sub-6-GHz frequencies and the mmWave frequencies. LOS battlefield radios take advantage of wide operating bandwidths but restrict signals to narrow portions of RF/microwave frequency spectrum compared to wireless cellular networks. Added infrastructure for LOS radio networks typically includes portable antenna towers and omnidirectional antennas that can be easily transported by troops and erected quickly as needed for networking capabilities (Figure 2).
[Figure 2 | Personnel place antennas for LOS battlefield radios during a training session. Photo credit U.S. Army.]
Sizing up a solution
Modern battlefield communications include many “things” in addition to troops and vehicles, notably IoT sensors. Spread throughout the varying terrain of a battlefield, wireless IoT sensors collect information about battle conditions like temperature and even the proximity of enemy assets. However, for such data to be useful, it must be communicated to a command center for analysis and distribution to troops in the field, and such communications must be secure to ensure the integrity of the collected data. Secure, wide-channel bandwidths are needed because of the substantial amounts and sensitivity of data to be transferred, both of which can be supported by LPI/LPD radio capability. To meet size, weight, and power (SWaP) goals, low current operation at a low, single-supply voltage enables long running times. This capability is the foundation of in-field embedded LPI/LPD LOS radios capable of maintaining reliable communications between IoT sensors, troops, and/or command centers.
Obviously, an LPI/LPD LOS radio is a complex design with circuitry becoming smaller and denser as designers seek to meet the defense world’s demands for reduced SWaP. The radios blend mixed-signal circuitry with firmware and software to send and receive information while remaining unnoticed by the enemy. But without encrypted signals, frequency hopping, and channel-changing capabilities – just to name a few – enemy EW equipment can listen to those signals as if they were their own. Constructing an effective LPI/LPD LOS radio requires many modules working together to achieve the required functionality, starting with the RF/microwave radio module, which must combine low-power operation with high RF performance in a small module size that enables embedding in portable, lightweight applications for long-term in-field use.
An example of a single-board low-power radio module from Benchmark Secure Technology measures 0.930 by 0.750 by 0.117 inches while securely communicating voice, compressed video, and data rates to 6.8 Mb/sec at distances to 200 m using six frequency bands from 3.5 to 7.0 GHz for frequency agility. The compact module includes a power supply and microprocessor with mesh ad-hoc, multihop networking capability. In environments where access to GPS signals for geolocation is not available, it is capable of two-way mesh-precision ranging with 10 cm accuracy supporting relative position location information (PLI). To support portable, battery-powered operation, the module runs on a single +3.3-VDC power supply. Depending on the channel-center frequency, it operates on instantaneous bandwidths of 500 and 900 MHz with transmit power of -14 dBm and transmit power density of less than -41.3 dBm/MHz. The castellated, self-contained PCB assembly module is a potential building block for advanced LPI/LPD LOS radios for terrestrial communications but can also be used for two-way radio systems that must operate within less-than-ideal propagation environments, like tunnels or bunkers.
These tactical LPI/LPD radios must be designed with extreme flexibility and adaptability to changing enemy capabilities and environmental conditions. Before being ready for in-field use, any LPI/LPD radio design is subjected to extensive system-level simulation, modeling, and testing under certain types of conditions (i.e., jamming and detecting signals) that it is expected to face in the field. Modules must be thoroughly tested for relevant military operational characteristics and meet SWaP requirements. For a battlefield of the future – crowded with signals as well as IoT sensors – it is the possible core of a secure radio link that will remain powered and ready for years in standby/sleep mode.
A graduate of California State University, Jim Benson currently works as a Director of Advanced Technology at Benchmark Electronics. Over the past nine years, Jim and his team have been working with the U.S. Army Research and Development organizations, developing low-power, low-cost solutions for soldier-borne body area and squad area data and voice communications. Mike Bontell is an aerospace and defense business development executive at Benchmark and a 20-year veteran of the United States Marine Corps, retiring with the rank of lieutenant colonel. Prior to joining Benchmark, Mike was a business leader at BAE Systems, L3Harris, and other defense systems providers. Hiep Truong is a Director of Design Engineering at Benchmark and is currently acting as a chief engineer for a variety of advanced wireless development projects. Prior to Benchmark, Hiep worked on system design and architecture development of wireless/SATCOM/VSAT systems for various military and government systems and commercial products. Hiep earned an MS and BS in electrical engineering from California State University.
Benchmark www.bench.com/secure-technology