Easing radar integration with SDR
StoryFebruary 20, 2023
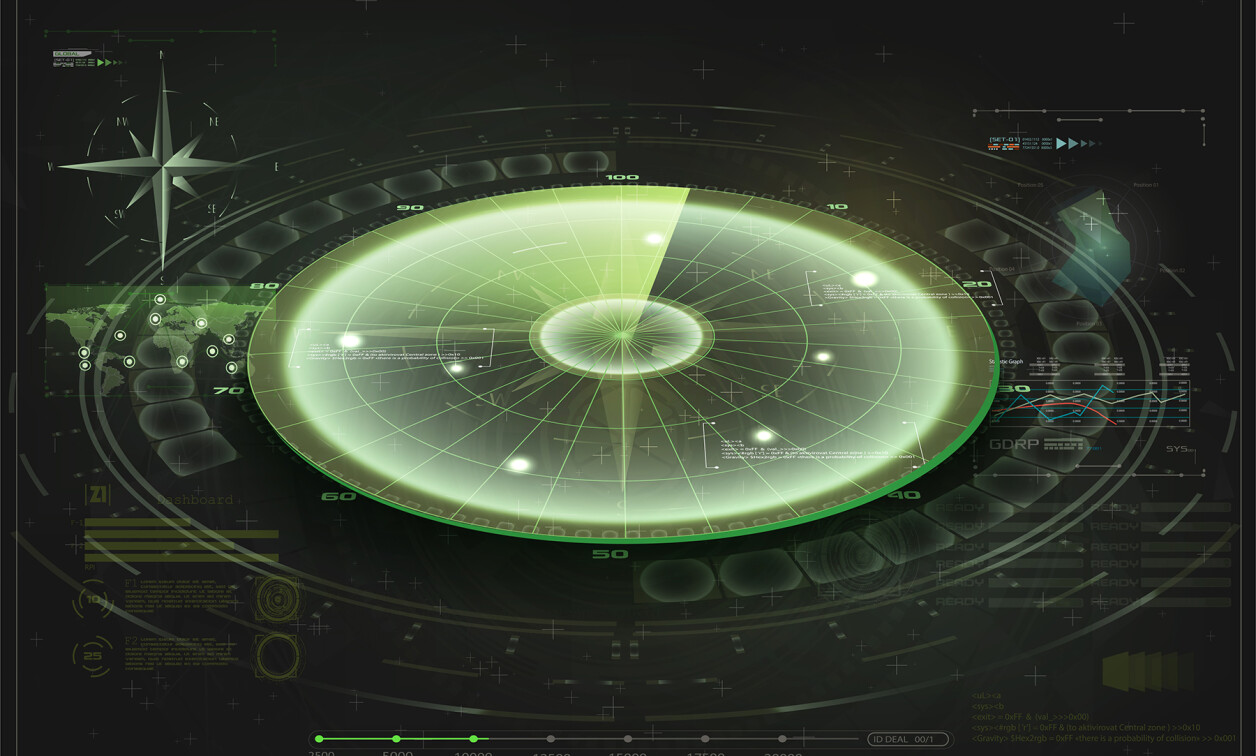
Software-defined radio (SDR) can assist with the integration and use of radar as it is used in electronic warfare (EW) systems and give users the flexibility to adapt to modern and constantly evolving threats.
Radar is widely applied in military missions, from field monitoring of defense systems and support devices for situational awareness to use in electronic countermeasures, guidance, and many other electronic warfare (EW) applications.
Although there are many types of radars, the basic principle is the same: An RF [radio-frequency] signal is sent in a certain direction, and if the signal hits an object, the reflected response is measured by a receiver to acquire spatial data. After signal reception, electrical parameters including signal amplitude, phase, frequency, and time delay can be used to infer the target’s distance, size, and speed. In this process, a computer host system must perform heavy-signal processing operations, which depend on the radar technique and application specifications. along with system control and display of measurements. By implementing a duplexer switch, radars can use the same antenna or dish for both signal transmission and reception, shifting between functions during the process.
Independently of the approach, synthetic aperture radar (SAR), Doppler, and phased-array systems rely greatly on heavy signal-processing algorithms, to manage the huge amounts of data required and control the antenna signal, which includes techniques such as beamforming and beam-steering.
Software-defined radios (SDRs) are RF devices that perform most of the radio- and digital-processing functions on the digital side, keeping analog equipment to a minimum. These devices are divided into two main functional blocks: the radio front end (RFE) and the digital back end. The RFE is responsible for all the analog functionalities required for the transmit and receive chains, including signal amplification, filtering, mixing, and antenna coupling. The RFE is a crucial part of the SDR, limiting its performance in terms of frequency, sensitivity, noise, and dynamic range. To address high-frequency applications, the highest-bandwidth SDRs in the market provide RFEs capable of providing several transmit/receive chains over a 0 to 18 GHz tuning range, that can be further upgraded to 40 GHz.
Multiple-input multiple-output (MIMO) capability can be achieved by implementing independent analog-to-digital converters/digital-to-analog converters (ADCs/DACs) at each RF channel of the RFE. The digital back end, on the other hand, handles all the digital signal processing (DSP) capabilities of the SDR, including modulation, demodulation, up/down-converting, and data packaging. It is typically implemented using a high-performance FPGA [field-programmable gate array], which enables parallel computation of simultaneous radio channels, minimizing latency and increasing data throughput. (Figure 1.)
[Figure 1 | Shown: an overview of the SDR board.]
How SDRs can be integrated into radar and EW systems
Regardless of the application and implemented technique, radar transceivers must fulfill a set of minimum requirements to ensure proper functioning and optimize use of resources.
The high-performance radio front end built in modern SDRs can be easily integrated into even the most complex and precise antenna system, providing a high degree of accuracy and flexibility to the radar. For one thing, high-end SDRs offer very wide tuning range in each channel, giving the user reliable channel spacing, the ability to use different radar protocols, and the option to use antijamming techniques. They provide receive signal chains with very low noise floor and high dynamic range, which improves the minimum and maximum detectable signals in the radar.
Up-to-date SDRs use multiple independent RF channels that can be tuned to different frequencies and can be simultaneously controlled and monitored over time. By integrating MIMO SDRs into antenna arrays, engineers can take advantage of their high phase and frequency stability to implement precision phased-array radars for EW applications and can program different beamforming/beam-steering algorithms to fit their application. High-end MIMO SDRs can offer as many as 16 channels in one device, each operating with an independent ADC/DAC for parallel acquisition. Naturally, radars implementing real-time monitoring of multiple channels require data-transfer systems with very high throughput, for further processing in the host units. To address this issue, the highest-throughput SDRs in the market offer quad 40 Gbps qSFP+ ports (upgradable to 100 Gbps) to handle the huge amounts of data involved. Figure 2 shows an SDR integrated into a radar system.
[Figure 2 | Diagram shows an SDR integrated into a radar system.]
The use of an SDR confers a clear hardware benefit, but the software-based signal processing is a bigger advantage to radar systems. High-performance SDRs use FPGA and DSP resources to perform very fast signal processing and parallel computations with very low latency, enabling the implementation of complex algorithms. Waveform digitization, with storage and triggering capabilities, is extremely important to a radar system, as it enables the radar to implement different waveforms in the same equipment while synchronizing components and sequentially triggering antenna arrays.
Sensitivity time control (STC) and sensitivity gain control (SGC) are examples of algorithms that can be embedded into the FPGA to precisely control the sensitivity of the radar to address power drops in the EM [electromagnetic] signal or saturation caused by unwanted signals. Finally, the DSP capabilities of the SDR can be used to implement complex mathematical algorithms, such as the fast Fourier transform (FFT), to analyze the frequency content of the signal and measure the Doppler shift, which can produce information about the velocity of the target. The ability to perform such algorithms in the radar itself is a huge advantage over conventional approaches, as much less data needs to be sent to the host system.
Beamforming and beam-steering are important for phased-array antennas, as they use multiple signals distributed in time (phase) and space to form a precise RF beam that can be pointed in a certain direction, therebv increasing the signal sensitivity and rejecting unwanted directions. The signal-processing algorithms are complex, requiring powerful computation resources to implement them fast enough to ensure phase coherency. These can actually be implemented in high-end FPGAs, which form the core of most SDRs. One of the most important steps in beam-steering is calculating the phase shift between channels. Figure 3 shows how to calculate this shift for a planar array of antennas, where the shift is a function of the distance between the radiating elements, the beam direction angle, and the wavelength of the signal.
[Figure 3 | Diagram shows a linear array of antennas and how the phase shift of each is calculated.]
Besides the functional advantages, SDRs can reduce the overall cost and complexity of a system: MIMO SDRs can perform many functions in the same device, which can dramatically lower the total equipment count in a system, which then reduces the maintenance and operation costs of the device and optimizes time to deployment. Further, SDRs are flexible enough to adapt to work with legacy radar systems; new EW deployments including electronic countermeasures and guidance; and service-life extension programs.
Kaue Morcelles is an electrical engineer, with emphasis on electronic design and instrumentation. He also works on developing instrumentation devices for tissue engineering. Morcelles works with Per Vices.
Per Vices https://www.pervices.com/