SiPs send clear signals for UAVs
StoryMay 11, 2023
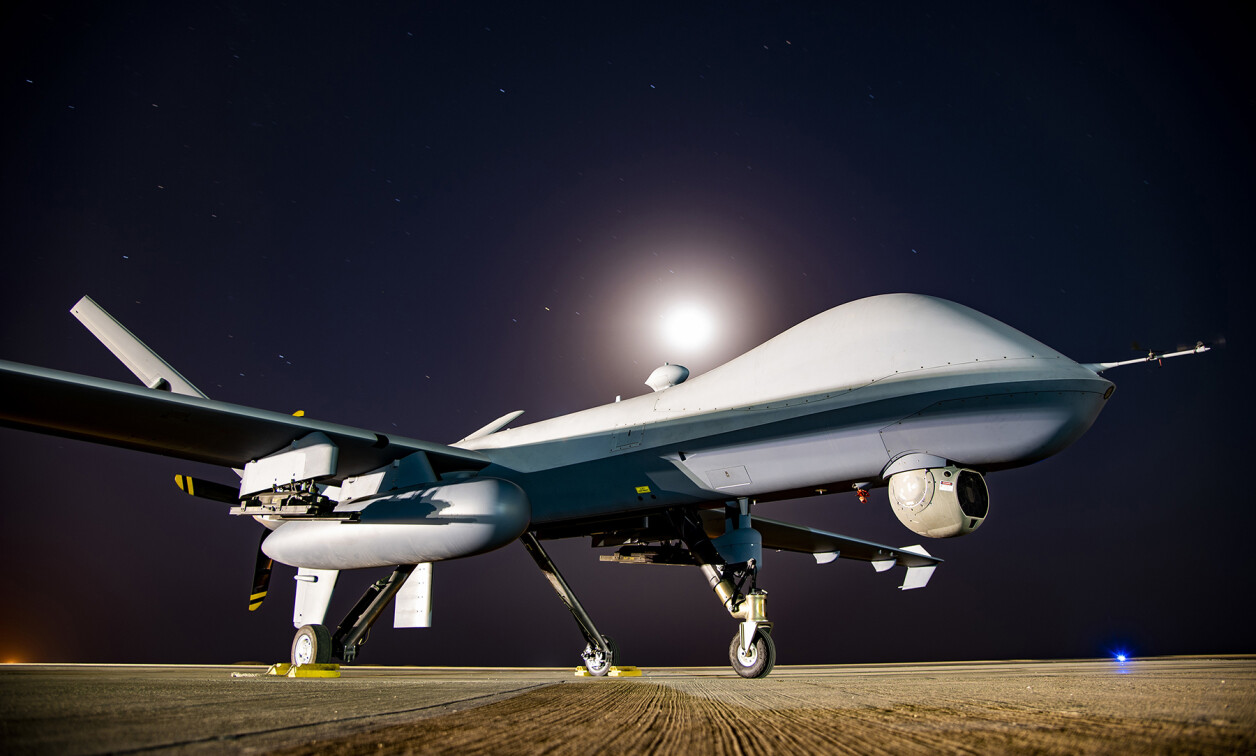
Unmanned aerial vehicles (UAVs) are rapidly swarming modern battlespaces, growing in number as they shrink in size. They are meeting strict guidelines for reduced size, weight, and power (SWaP) even as their capabilities increase, packing payloads that include offensive ordnance, transmitters, receivers, cameras, and sensors. Miniaturization contributes not only to added functionality but to longer flight times and ranges on a battery. Advancing UAV technology for the battlefield presents the ongoing challenge of developing electronic payloads that are smaller and with wider bandwidths to support multiple sensors without delays. What once was a complete electronic warfare (EW) system carried by a large ground vehicle must now fit within a flight-ready vehicle that, in some cases, can be launched by hand. Fortunately, by fitting multifunction circuitry within a single multipin package, in the form of system-on-chip (SoC) or system-in-package (SiP) devices, versatile payloads can be designed to make smaller, more capable military UAVs possible.
Payloads for a medium-sized unmanned aerial vehicle (UAV) or drone combine numerous technologies in support of armaments, communications, guidance and navigation, reconnaissance, and surveillance, among other functions. Electronic systems within those payloads often merge various electro-optical subsystems, such as radar, light detection and ranging (Lidar), and infrared (IR) thermal sensing subsystems, within these payloads. Antennas and possibly sensors are usually mounted in the front or nose of a UAV while the remainder of the electronic systems are spread throughout the body of the aircraft.
What was once designed and assembled on multiple printed circuit boards (PCBs) mounted within multiple equipment enclosures to fit within the payloads of full-sized aircraft must now provide the same functionality, often with improved performance, at a fraction of the size in efforts to meet military goals for reduced size, weight, and power (SWaP). Modular design has been one of the evolutionary steps in reducing the SWaP of UAV electronic payloads, by partitioning functionality into compact, low-power modules that can then be interconnected within a UAV’s payload to provide the functions and performance needed for that particular drone design. However, as tactical drones continue to shrink in size, the modular approach cannot keep pace with the diminishing size and weight requirements of, for example, hand-launched drones (Figure 1). Rather than continuing to design UAV payloads as multiple modules, a single enclosure must be developed capable of providing all the functionality and performance required.
[Figure 1 | U.S. Army Pfc. Austin Hurt (1st Battalion, 26th Infantry Regiment, 2nd Brigade Combat Team, 101st Airborne Division [Air Assault]), throws an RQ-11B Raven unmanned aerial vehicle into the sky of a forward operating site in southeastern Europe. These soldiers are using the Ravens to spot targets, support call-for-fire missions, and in many other reconnaissance tasks when conditions are not favorable for manned missions. (Photo credit: U.S. Army Pfc. Matthew Wantroba.)]
Higher circuit integration contributes to reducing UAV payload SWaP but components and modules in close proximity to radiating components, such as oscillators, can pose electromagnetic interference (EMI) and electromagnetic compatibility (EMC) challenges in circuit and system layouts. Computer modeling and simulation can provide invaluable insights into circuit and layout designs prior to prototyping and EMI/EMC testing. Multifunction devices such as system-on-chip (SoC) or system-in-package (SiP) designed for high-density layouts can integrate shielding within packages for minimal EMI/EMC problems.
Small aircraft, large performance
Even as military drones pack more features into smaller aircraft, with growing numbers of sensors, they are also expected to reach new performance levels. Achieving smaller UAVs with greater functionality requires the signal-processing capabilities usually found in much larger aircraft, and processing capability embedded in FPGAs and ASICs is needed to manage data from a wide range of sensors, including IR thermal sensors, radar altimeters, and visible-light cameras. The resolution and accuracy of modern IR thermal sensors is such that they can help land a UAV in a docking station without human direction. As part of obstacle detection subsystems, Lidar sensors help military UAVs avoid powerlines and other flight impediments.
Reliable communications – by means of air-to-air, air-to-ground, and satellite communications – are needed not only to transfer sensor data from a UAV to its ground-control station, but also to provide remote control. A UAV’s onboard communications receivers and transmitters must enable guidance and navigation, such as by reception of signals from GPS satellites.
The amount of lag time permissible in a UAV’s communications links depends upon the function being accessed. Short lag times can be tolerated in regular sharing of data from the many onboard sensors, such as the visible-light cameras performing routine surveillance. For control of an on-board armament system, such as a short-range missile or projectile or even a laser-based weapons system, instantaneous synchronization of several systems is required with high-resolution imaging supporting precise targeting. Such high-speed communications capabilities at high data bit rates imply wide channel bandwidths. Since spectrum tends to be occupied at lower RF and microwave frequencies, UAV payload designers are increasingly looking to higher frequencies for available wide-bandwidth channels to carry the immense amounts of data collected by a tactical drone’s sensors.
The large numbers of sensors, the use of uncharted wireless frequency bandwidths, the rapid analog-to-digital signal processing required, and the overall large amounts of on-board, in-flight computing power needed for autonomous and remote control of a tactical UAV all point to large electronic payloads with multiple modular subsystems providing performance reliable enough to withstand the environmental operating guidelines for military and aerospace applications. The control systems for tactical UAVs and other autonomous military electronic systems must be flexible and scalable since, depending upon the mission, the size of a UAV can range from as small as a hawk to as large as a helicopter capable of transporting troops and armaments.
Developing UAV electronic payloads in step with reduced SWaP goals calls for circuit and device densification and miniaturization unlike anything that has been possible even with multiple ICs. Higher device density is needed, by fabricating complete subsystems that can fit within a surface-mount-technology (SMT) package. In contrast to ICs, which typically provide one or two components such as amplifiers and switches plus impedance-matching circuitry within a single drop-in package, a transceiver or mixed-signal front-end SoC or SiP can fit in a single package. By adopting a SiP-based strategy, a UAV payload designer can essentially combine all the subsystems needed one package at a time, to create a multiple-function electronic payload that can fit within the limited space of a tactical drone complying with reduced SWaP requirements.
An SiP is not the same as an SoC, although they may represent the same potential savings in board space for a UAV circuit designer. A SiP houses all electronic devices, which may include multiple ICs, microcontrollers, and discrete devices, within a single multipin package. An SoC fabricates the multiple functions on the same chip or monolithic circuit. The advantage of SiPs over SoCs is the opportunity to mix semiconductor processes (e.g., silicon radio-frequency ICs [RFICs] and gallium arsenide [GaAs] amplifiers) and discrete components (e.g., filters, ferrites, and inductors) in a single envelope. An SoC can provide a great deal of functionality with high yield while an SiP excels at providing optimum performance while minimizing application sensitivity at the next higher assembly.
What does an SiP do?
What types of subsystem functions can be provided by SiPs? At present, some SiPs are designed, manufactured, and tested according to the electrical, environmental, and mechanical requirements of military and aerospace applications. They provide the communications bandwidth for essential electronic-warfare (EW) systems – from 6 GHz to 18 GHz – as well as the extended-frequency bandwidth, within the millimeter-wave frequency range, to channel large amounts of data from the many onboard sensors as quickly as possible to in-air, at-sea, and ground-based end users whose lives may literally depend on the data.
One path to reduced SWaP is by replacing legacy defense subsystems with solid-state modules and devices, such as SoCs and SiPs. Miniaturization is also possible for electromechanical systems in UAVs, such as the gyroscopes and accelerometers needed for guidance and control when GPS signals may be blocked or jammed. Size can be reduced by applying microelectromechanical system (MEMS) strategies.
As an example of the evolution taking place in monolithic device technology, the ADTR1107 from Analog Devices is a packaged IC, a front-end subsystem with frequency range of 6 to 18 GHz. (Figure 2.) It is designed to raise the levels of signals from a UAV’s EW transmitter to its antennas and to boost signals from the antennas to the EW receiver with minimal noise. It replaces what would have been ICs for a power amplifier (PA), low-noise amplifier (LNA), and an antenna switch between the two amplifiers. This single device saves space on a circuit board by enclosing the three components and their interconnections and power-supply connections in a 24-terminal land-grid-array (LGA) package measuring 5 mm by 5 mm.
[Figure 2 | The model ADTR1107 is a 6 GHz to 18 GHz EW receiver front-end IC in a package measuring 5 by 5 mm.]
In support of the phased-array antennas typically used for tactical drones, the model ADAR1000 places a four-channel beamformer into an 88-terminal LGA package that is only 7 mm by 7 mm and specified for -40 °C to +85 °C (Figure 3). Fabricated with a silicon-germanium (SiGe) BiCMOS [bipolar CMOS] semiconductor process, it operates from 8 GHz to 16 GHz with a 360-degree phase adjustment range and 2.6-degree phase resolution. It features single-pin transmit/receive control, which also aids in synchronizing multiple devices, and enough memory to store 121 beam positions. The device is power efficient, with all on-chip registers controlled by a simple four-wire serial peripheral interface (SPI).
[Figure 3 | Model ADAR1000 is a four-channel, 8 GHz to 16-GHz SiGe BiCMOS beamformer in a 7 × 7 mm LGA package.]
High levels of integration result in appreciable power savings as size and weight are being minimized. In addition, with the advanced power control possible in an SoC or SiP, the high power efficiency translates into extended range per battery charge for a UAV.
UAVs have been part of the battlefield for as long ago as the Vietnam War; during that conflict they were used for reconnaissance. They are replacing living troops in high-threat environments even as the latest wave of UAVs is being referred to as “uninhabited combat air vehicles” (UCAVs) by several of the major defense contractors. While drones typically have a flight time of under one hour on internal battery power, evolving UAV technology is seeking longer-endurance UAVs that can make use of solar power. Tactical drones are also making more use of artificial intelligence (AI) for coordinated efforts with manned aircraft. The Boeing MQ-28 Ghost Bat, initially part of the Loyal Wingman project, is an example of a highly “intelligent” military UAV. Under development by Boeing Australia for the Royal Australian Air Force (RAAF), it is being equipped with sufficient AI and tactical capabilities to perform autonomous missions and to fly alongside and support manned aircraft in battle.
As part of the ongoing evolution of battlefield drones, a great deal of technology-sharing occurs between military and commercial UAV supplies, since commercial UAV markets are projected to grow rapidly once government agencies such as the Federal Aviation Administration (FAA) have set acceptable guidelines for their commercial and industrial use. Demand for applications in markets such as warehouse inventory control and monitoring are expected to be strong for decades. Providing adequate electronic payloads in small enclosure sizes will require highly integrated device solutions that can fit a system on an SoC or within a single package, such as in a SiP.
Jerome Patoux is a director for the Aerospace and Defense Business Unit, with 18 years of experience in the semiconductor industry. He is currently with Analog Devices, Inc. (ADI), based in Wilmington, Massachusetts. Jerome holds a master’s degree in electrical engineering and RF communications from EFREI-ESIGETEL Engineering University in Paris, France, and a Master of International Program Management from the University of Quebec in Ottawa-Gatineau, Canada and from ISMANS, Superior Institute for Materials and Advanced Mechanics in Le Mans, France. Jerome started his career as a field application engineer with a specialty in power-management products and solutions, transitioning to various product marketing and product-line management roles. The author can be reached at [email protected].
Analog Devices, Inc. • https://www.analog.com/